Neural Regeneration
Biomedical Devices
Assistive Devices
Soft Robotics
Biomaterials
Bio-printing
Wearables
Neuroprosthetics
Sensors
Student Highlights
Neural Regeneration
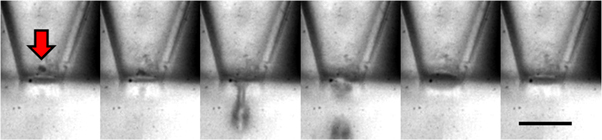
Printing of single cells
E. Cheng, H. Yu, A. Ahmadi, and K. C. Cheung, Investigation of the hydrodynamic response of cells in drop on demand piezoelectric inkjet nozzles, Biofabrication 2016 8, 015008
Single cell printing is being developed using piezoelectrically actuated inkjet printing towards cell printers for tissue engineering and fine-control over heterogeneous tissue structure at the interface with artificial materials.
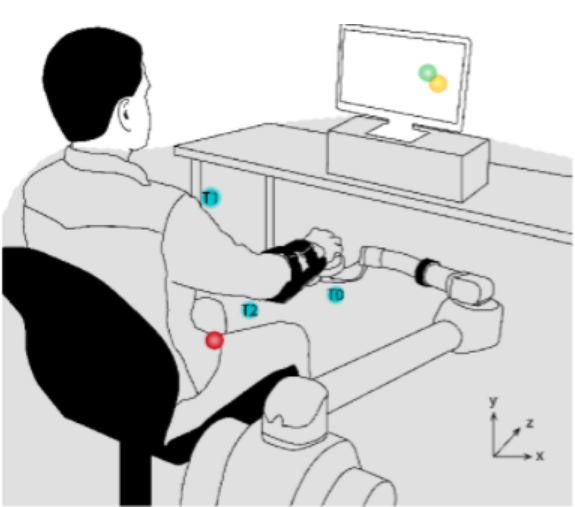
Robot-guided recovery of proprioception
B. A. Valdes, M. Khoshnam, J. L. Neva, C. Menon, Robot-aided upper-limb proprioceptive training in three-dimensional space, IEEE 16th International Conference on Rehabilitation Robotics (ICORR), 2019, 18920185
Robotic devices can be used to track and facilitate arm movements, and therefore can help establish recovery protocols aimed at enhancing proprioception.
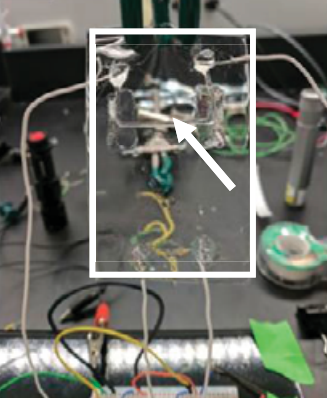
Building an artificial peripheral nerve sensor
C. Hamilton, K. Tian, J. Bae, C. Yang, G. Alici, G. M. Spinks, Z. Suo, J. J. Vlassak, and M. in het Panhuis, “A Soft Stretchable Sensor: Towards Peripheral Nerve Signal Sensing,” MRS Advances, vol. 3, no. 28, pp. 1597–1602, 2018
Dr. in het Panhuis’s research in soft robotics draws inspiration from biological systems to 3D print structures that enhance human aquatic mobility and build soft structures that sense human nerves.
Biomedical Devices
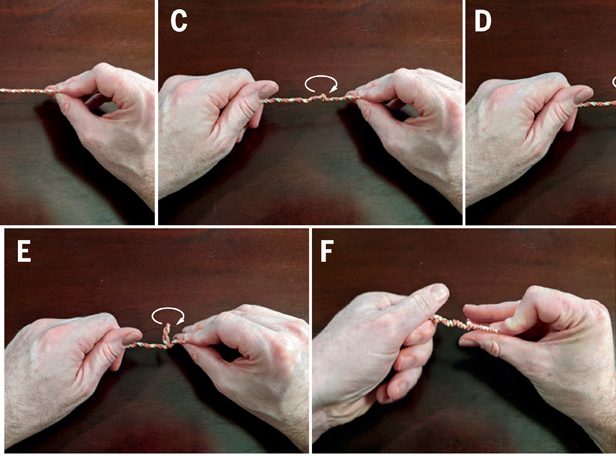
Unraveling DNA inspires artificial muscle
Madden, J.D.W. 2021, "Unraveling DNA inspires artificial muscle", Science robotics, vol. 6, no. 53.
Assistive Devices
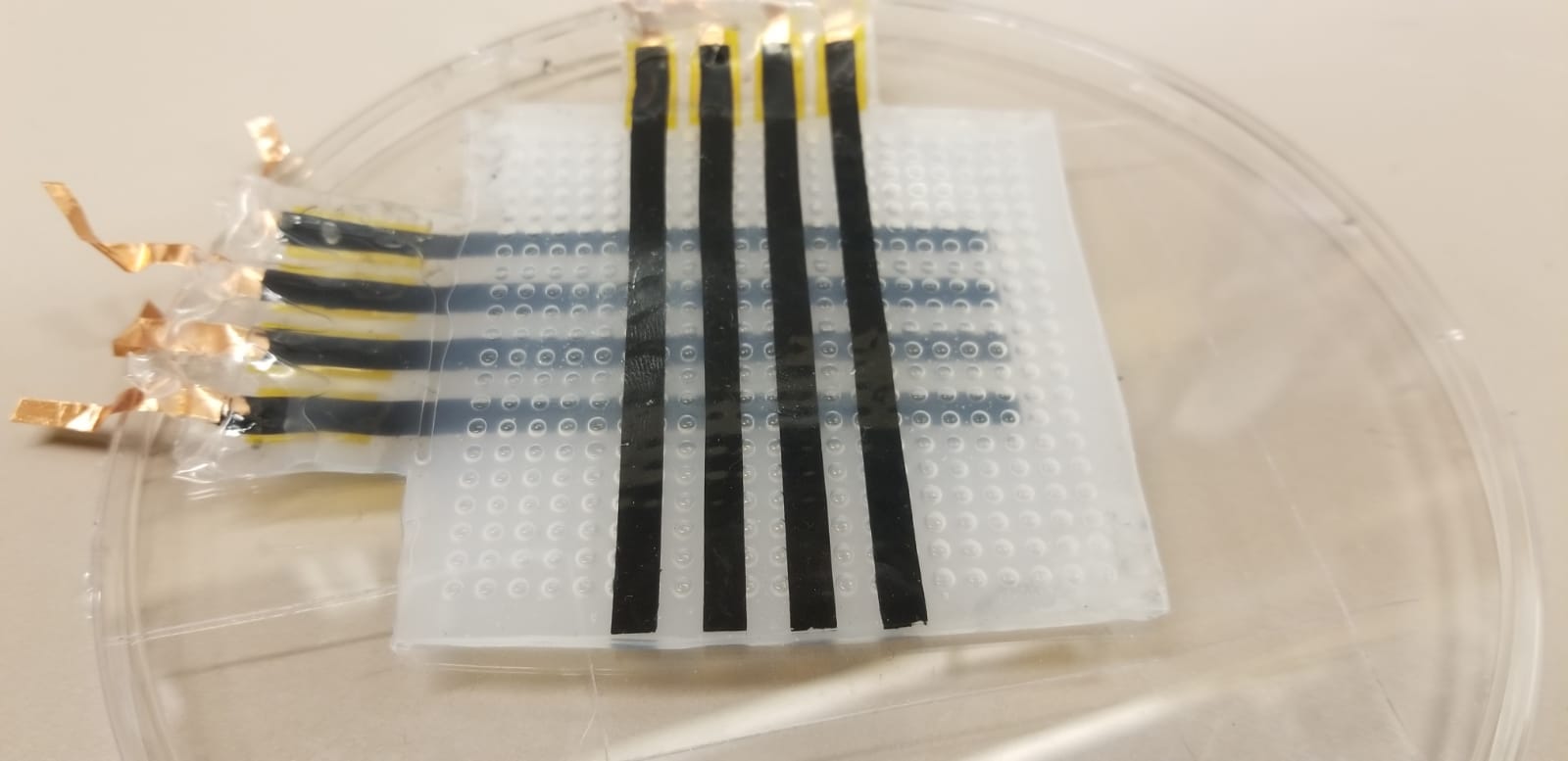
Pressure detection device
J. D. W. Madden et al., Proximity and touch sensing using deformable ionic conductors, Proceedings Volume 10163, Electroactive Polymer Actuators and Devices (EAPAD) 2017, 2017, 1016305
The Madden lab is developing a prevention pressure-sensing mat for pressure ulcer prevention, by detecting pressure, proximity and touch during active deformation of a surface, for integration into bed sheets to detect pressure points.
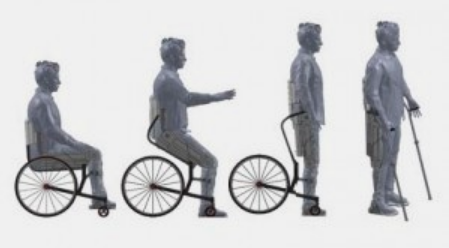
Building technology to increase autonomy of spinal cord injury population
M. Khalili, H. F. M. Van der Loos, J. Borisoff, Towards and autonomy-based approach to design and develop mobility assistive technologies for spinal cord injury population 2018, Rehabilitation Engineering and Assistave Technology Society of North America Conference
Mobility impairment is the third most common form of disability in Canada, and a large number of the mobility impared population relies on wheeled mobility devices, but classical wheeled devices are limited in their range of autonomy. By incorporating new designs, device-specific restrictions on mobility can be addressed to give the user more autonomy.
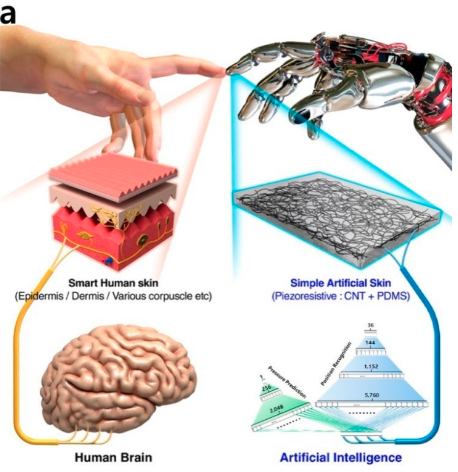
Tactile sensor technology and smart tactile sensing systems
L. Zou, C. Ge, Z. J. Wang, E. Cretu, X. Li, Novel tactile sensor technology and smart tactile sensing systems: A review, Sensors 2017, 17(11), 2653
Dr. Cretu’s lab is focused on developing bio-medical devices through research of adapted microsystems and microstructures. They are looking for impactful ways to use his research with tactile sensors to create advanced biofeedback devices.
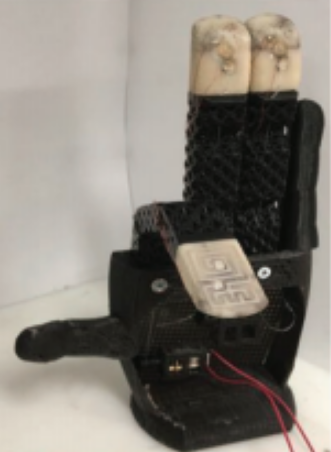
Human-like movement of fingers stimulated by bionic taste
T. Kim, M. Kaur, W. S. Kim, Humanoid Robot Actuation through Precise Chemical Sensing Signals, Advanced Materials Technologies, 2019, 4, 1900570
Kim's lab integrates a taste sensor for salts in the tips of robotic fingers that bend to various degrees when they encounter different ion concentrations.
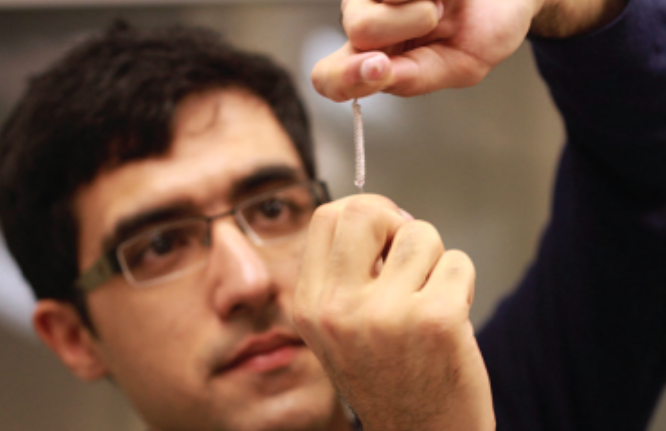
Artificial muscles
C. S. Haines, M. D. Lima, G. M. Spinks, J. Foroughi, J. D. W Madden, et al., Artificial Muscles from Fishing Line and Sewing Thread, Science 2014, Vol. 343, Issue 6173, pp. 868-872
The Madden lab is developing an artificial muscle made of nylon that has similar contraction length to human muscle, but 100x stronger, with potential application in biomedical and robotic devices.
Soft Robotics
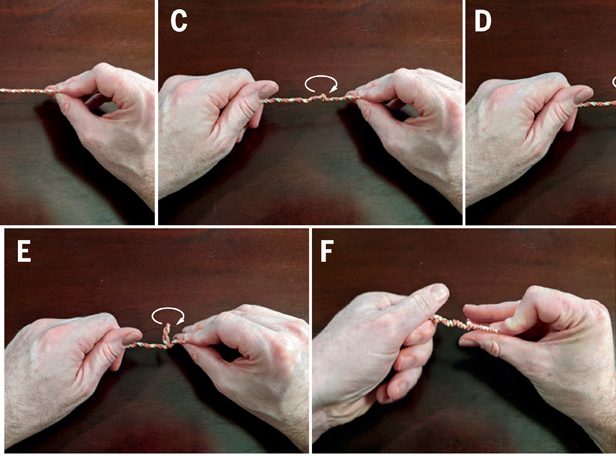
Unraveling DNA inspires artificial muscle
Madden, J.D.W. 2021, "Unraveling DNA inspires artificial muscle", Science robotics, vol. 6, no. 53.
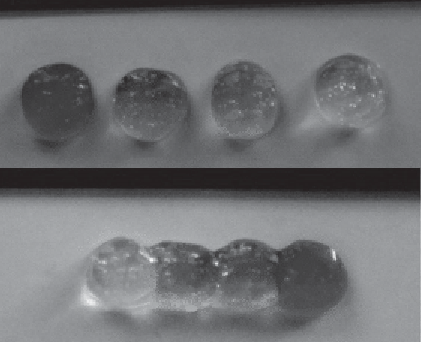
Autonomously self-healing hydrogels
D. L. Taylor, M. In Het Panhuis, Self-Healing Hydrogels, Adv Mater. 2016, 28(41), 9060-9093
Self healing hydrogels facilitate soft, deformable materials and components that can confirm to complex and changing environments such as biological interfaces and can regain shape and functionality after large deformations.
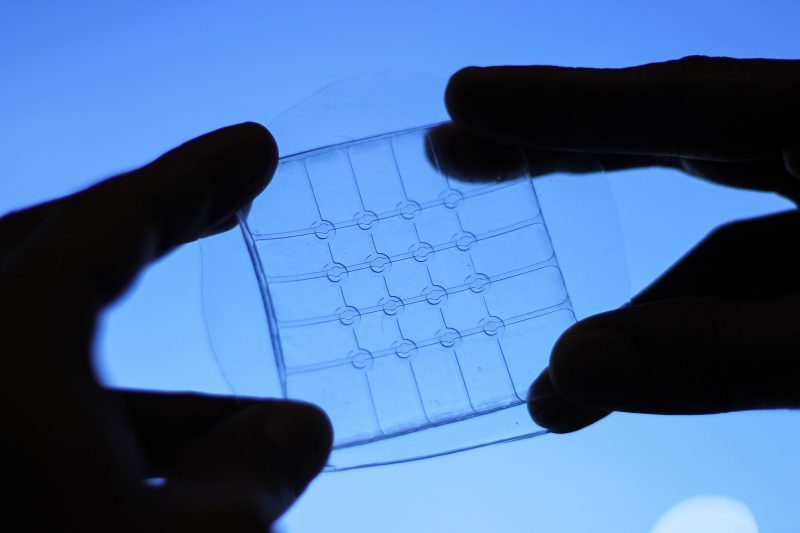
Transparent sensor array
M. S. Sarwar, Y. Dobashi, C. Preston, J. K. M. Wyss, S. Mirabbasi, J. D. W. Madden, Bend, stretch, and touch: Locating a finger on an actively deformed transparent sensor array. Science Advances 2017, 3, e1602200
John Madden's research team has created a stretchable transparent soft tactile sensing skin towards flexible wearables and humanoid soft robotics
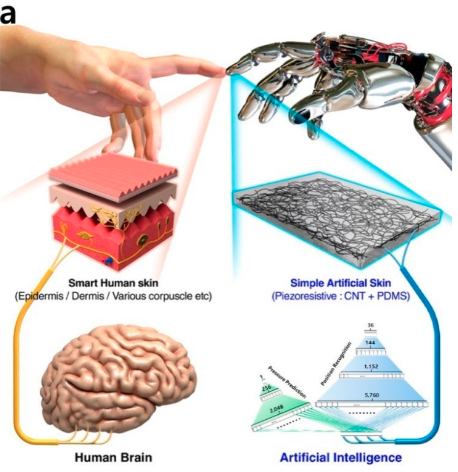
Tactile sensor technology and smart tactile sensing systems
L. Zou, C. Ge, Z. J. Wang, E. Cretu, X. Li, Novel tactile sensor technology and smart tactile sensing systems: A review, Sensors 2017, 17(11), 2653
Dr. Cretu’s lab is focused on developing bio-medical devices through research of adapted microsystems and microstructures. They are looking for impactful ways to use his research with tactile sensors to create advanced biofeedback devices.
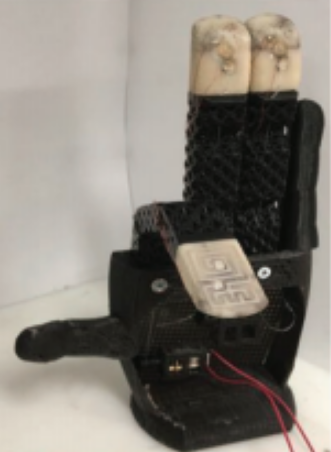
Human-like movement of fingers stimulated by bionic taste
T. Kim, M. Kaur, W. S. Kim, Humanoid Robot Actuation through Precise Chemical Sensing Signals, Advanced Materials Technologies, 2019, 4, 1900570
Kim's lab integrates a taste sensor for salts in the tips of robotic fingers that bend to various degrees when they encounter different ion concentrations.
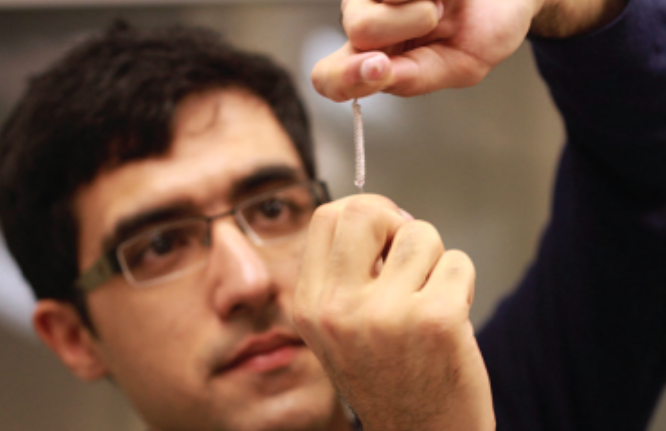
Artificial muscles
C. S. Haines, M. D. Lima, G. M. Spinks, J. Foroughi, J. D. W Madden, et al., Artificial Muscles from Fishing Line and Sewing Thread, Science 2014, Vol. 343, Issue 6173, pp. 868-872
The Madden lab is developing an artificial muscle made of nylon that has similar contraction length to human muscle, but 100x stronger, with potential application in biomedical and robotic devices.
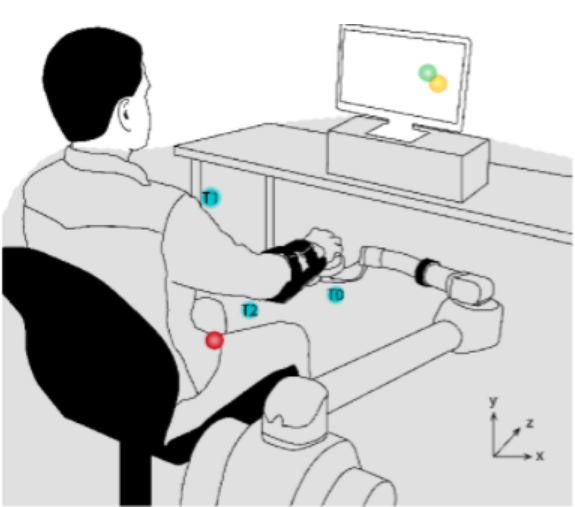
Robot-guided recovery of proprioception
B. A. Valdes, M. Khoshnam, J. L. Neva, C. Menon, Robot-aided upper-limb proprioceptive training in three-dimensional space, IEEE 16th International Conference on Rehabilitation Robotics (ICORR), 2019, 18920185
Robotic devices can be used to track and facilitate arm movements, and therefore can help establish recovery protocols aimed at enhancing proprioception.
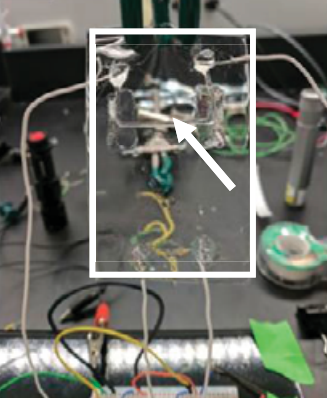
Building an artificial peripheral nerve sensor
C. Hamilton, K. Tian, J. Bae, C. Yang, G. Alici, G. M. Spinks, Z. Suo, J. J. Vlassak, and M. in het Panhuis, “A Soft Stretchable Sensor: Towards Peripheral Nerve Signal Sensing,” MRS Advances, vol. 3, no. 28, pp. 1597–1602, 2018
Dr. in het Panhuis’s research in soft robotics draws inspiration from biological systems to 3D print structures that enhance human aquatic mobility and build soft structures that sense human nerves.
Biomaterials
Bio-printing
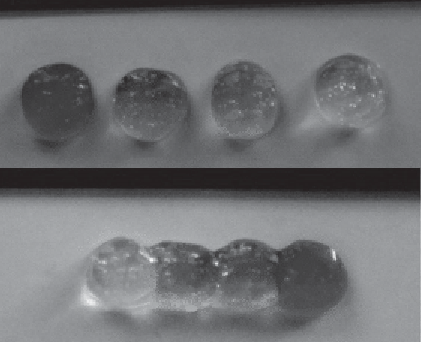
Autonomously self-healing hydrogels
D. L. Taylor, M. In Het Panhuis, Self-Healing Hydrogels, Adv Mater. 2016, 28(41), 9060-9093
Self healing hydrogels facilitate soft, deformable materials and components that can confirm to complex and changing environments such as biological interfaces and can regain shape and functionality after large deformations.
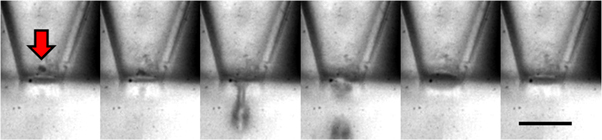
Printing of single cells
E. Cheng, H. Yu, A. Ahmadi, and K. C. Cheung, Investigation of the hydrodynamic response of cells in drop on demand piezoelectric inkjet nozzles, Biofabrication 2016 8, 015008
Single cell printing is being developed using piezoelectrically actuated inkjet printing towards cell printers for tissue engineering and fine-control over heterogeneous tissue structure at the interface with artificial materials.
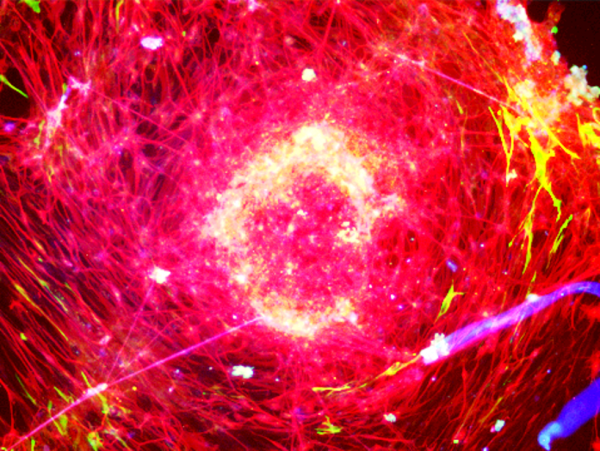
3D bio-printing neural tissue
L. De la Vega, K. Karmirian, S. M. Willerth, Engineering Neural Tissue from Human Pluripotent Stem Cells Using Novel Small Molecule Releasing Microspheres, Advanced Biosystems 2018, 2, 1800133
The Willerth lab is designing bio-printed heterogeneous tissue constructs to develop spinal cord-mimicking tissue with novel drug delivery systems using microparticles.
Wearables
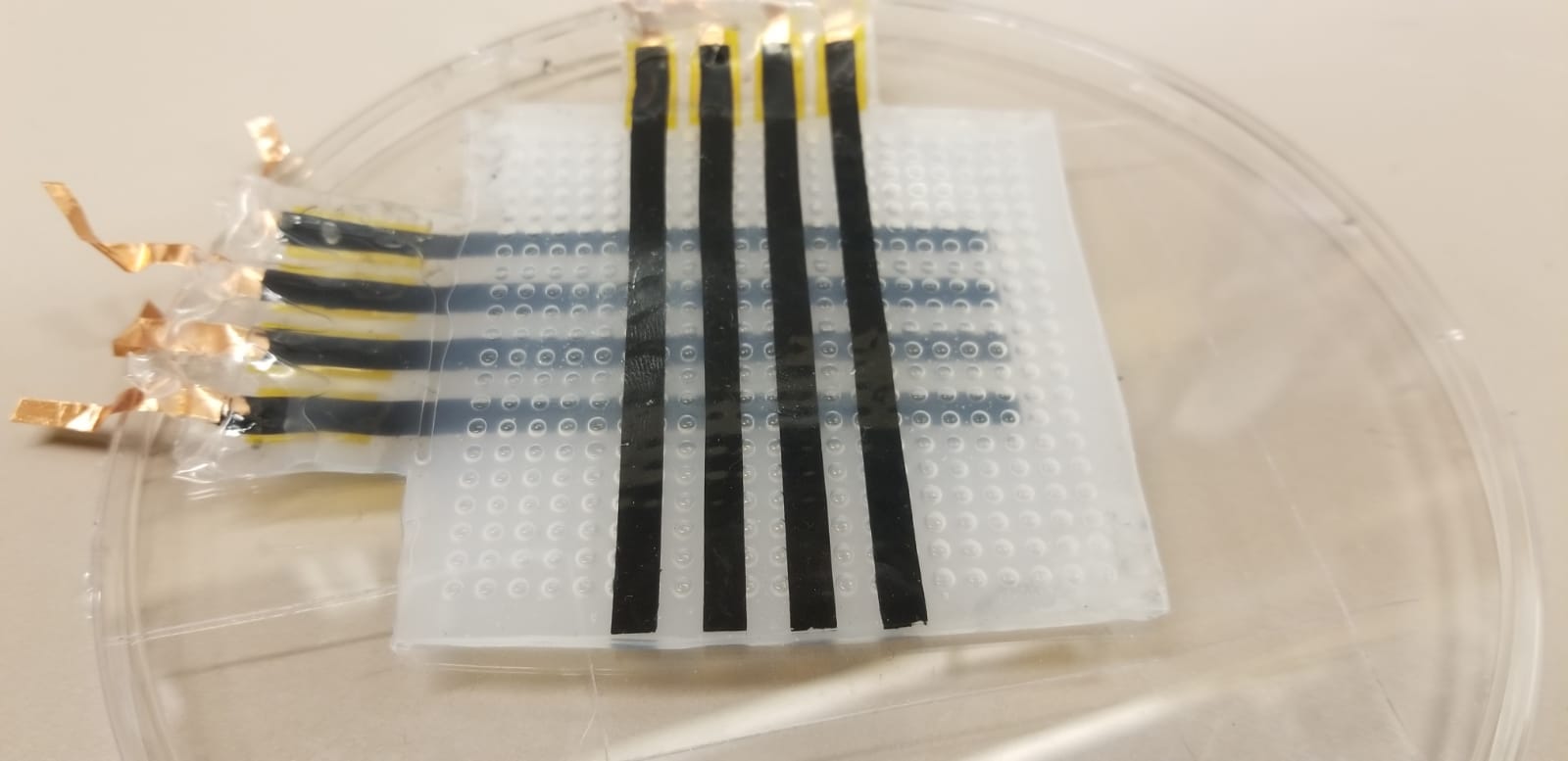
Pressure detection device
J. D. W. Madden et al., Proximity and touch sensing using deformable ionic conductors, Proceedings Volume 10163, Electroactive Polymer Actuators and Devices (EAPAD) 2017, 2017, 1016305
The Madden lab is developing a prevention pressure-sensing mat for pressure ulcer prevention, by detecting pressure, proximity and touch during active deformation of a surface, for integration into bed sheets to detect pressure points.
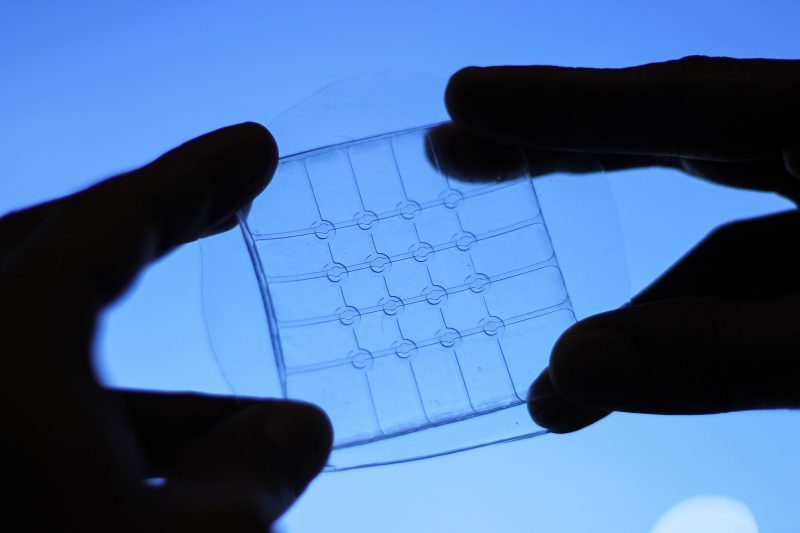
Transparent sensor array
M. S. Sarwar, Y. Dobashi, C. Preston, J. K. M. Wyss, S. Mirabbasi, J. D. W. Madden, Bend, stretch, and touch: Locating a finger on an actively deformed transparent sensor array. Science Advances 2017, 3, e1602200
John Madden's research team has created a stretchable transparent soft tactile sensing skin towards flexible wearables and humanoid soft robotics
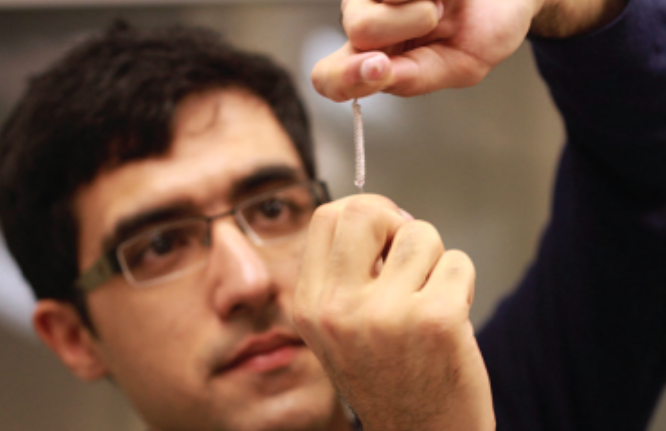
Artificial muscles
C. S. Haines, M. D. Lima, G. M. Spinks, J. Foroughi, J. D. W Madden, et al., Artificial Muscles from Fishing Line and Sewing Thread, Science 2014, Vol. 343, Issue 6173, pp. 868-872
The Madden lab is developing an artificial muscle made of nylon that has similar contraction length to human muscle, but 100x stronger, with potential application in biomedical and robotic devices.
Neuroprosthetics
Sensors
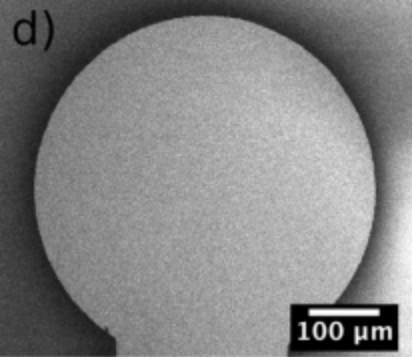
Coating gold electrodes with graphene for biological interfaces
Y. Li, I. Martens, K. C. Cheung, and D. Bizzotto, Electrodeposition of reduced graphene oxide onto gold electrodes: creating thin electrochemically active and optically transparent overlayers, Electrochimica Acta, 2019, 319, 649-656
Karen’s lab works on a variety of sensors to probe biological systems.
Student Highlights
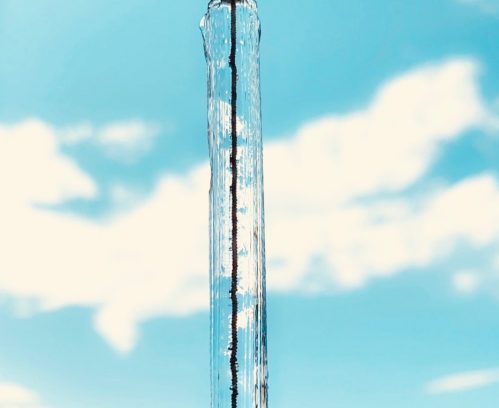
Smart sock for deep vein thrombosis
Sukhneet Dhillon
PhD, Biomedical Engineering
Sukhneet is developing coating and optimizing nylon artificial muscles for application to invasive and non-invasive medical devices as well as compression socks for patients with deep vein thrombosis.
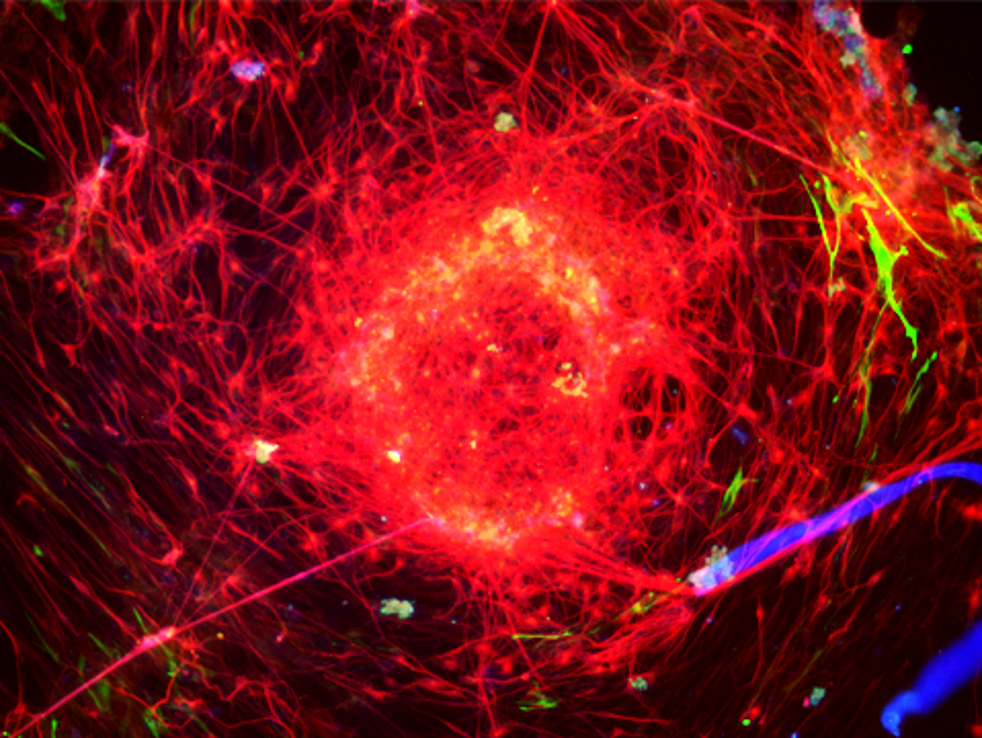
Bio-printing neural tissue
Laura de la Vega
PhD, Biomedical and Tissue Engineering
Laura has been developing 3D printed neural tissue with novel drug delivery systems.
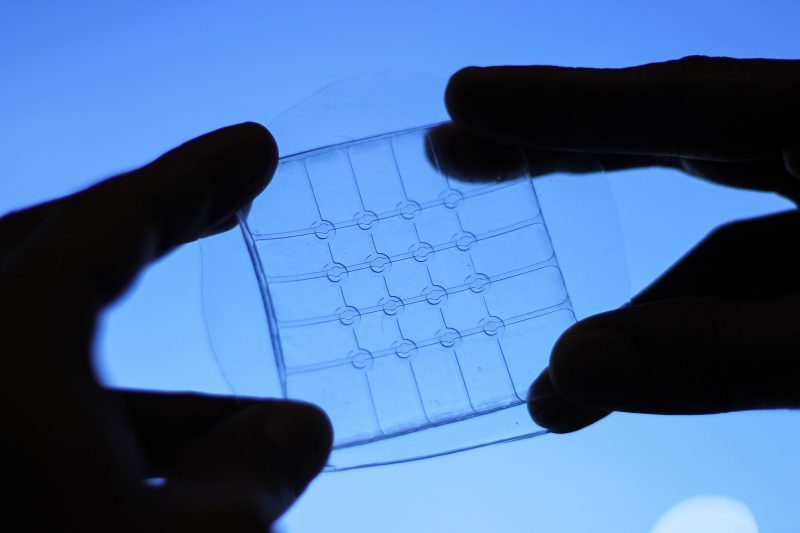
Transparent tactile sensors
Mirza Saquib Sarwar
PhD, Electrical and Computer Engineering
Saquib has recently published a new transparent touch sensor applicable to wearables and robotics that is flexible, stretchable and operates during active deformation.
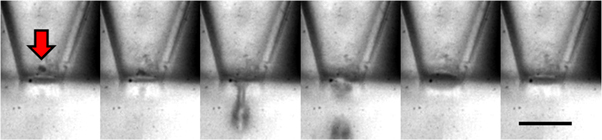
Inkjet cell printing
Eric Cheng
PhD, Biomedical Engineering
Eric has been developing technology to perform single cell printing using piezoelectrically actuated inkjet printing towards cell printers for tissue engineering and fine-control over heterogeneous tissue structure at the interface with artificial materials.

Nylon-based artificial muscle fibres
Xu Fan, Kieran Morton
BASc, Electrical and Computer Engineering
Fan and Kieran have been developing artificial muscle technologies towards higher strength, actuation speed and lower energy usage for biomedical assistive devices.
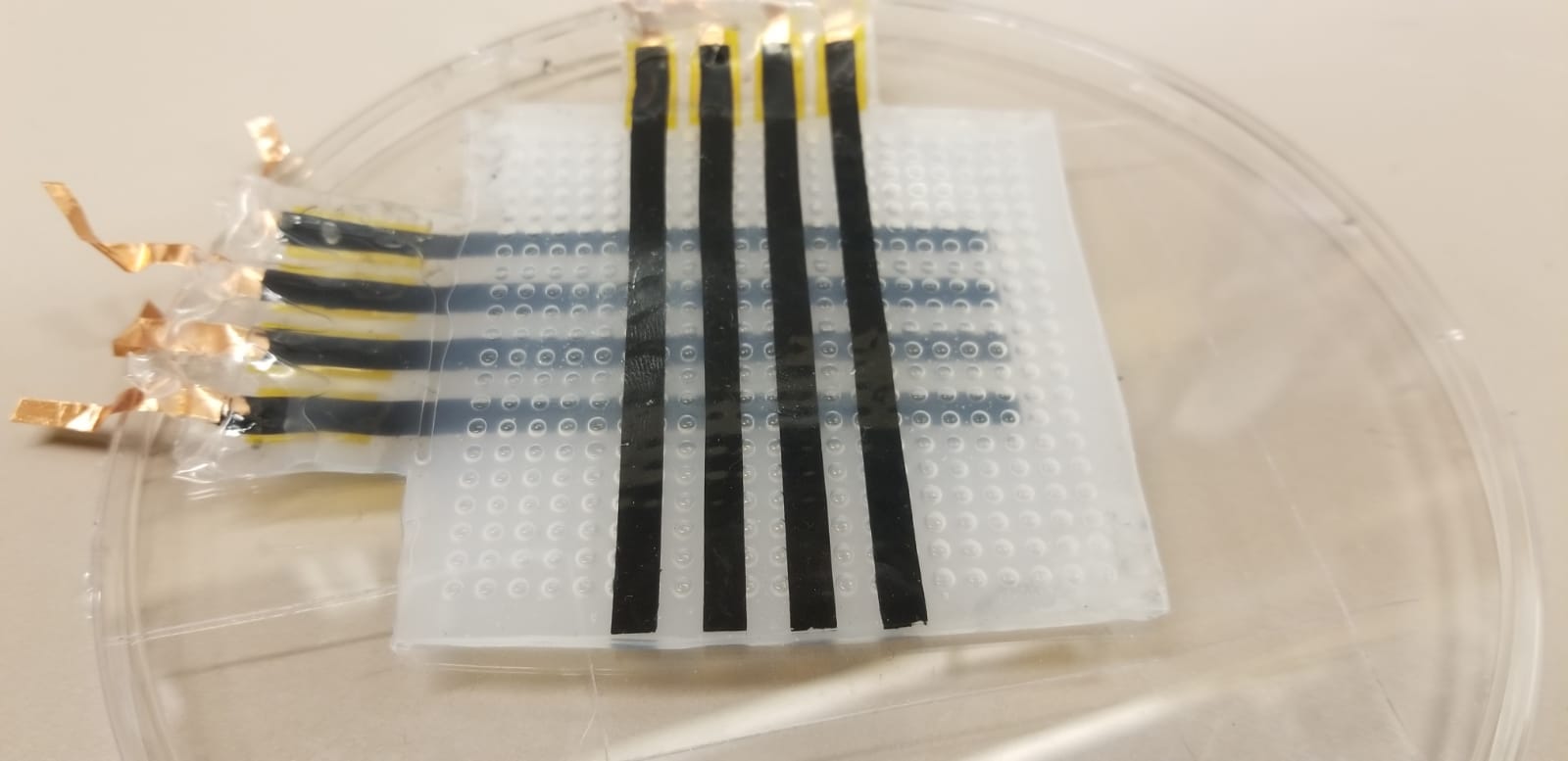
Prevention device for pressure ulcers
Justin Wyss
MASc, Biomedical Engineering
Justin is developing a prevention device for pressure ulcers, which are one of the most expensive preventable secondary complications for patients with spinal cord injury, using a new sensor technology that that detects proximity, touch and pressure.
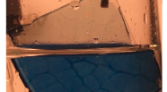
Stretchable electrochromic displays
Claire Preston
MASc, Electrical and Computer Engineering
Claire is developing stretchable electrochromic displays that change colour with application of voltage with application to wearable electronics for biomonitoring and camouflage as well as soft robotic skin.